Pipes Two Million Times Smaller Than an Ant
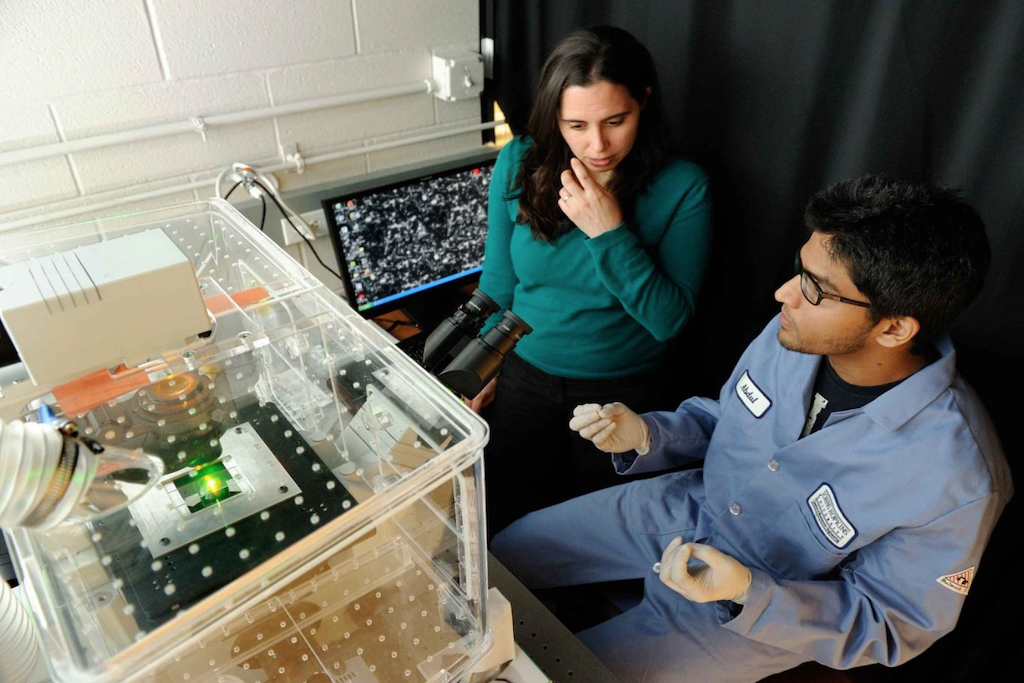
World’s tiniest plumbing could one day funnel drugs to individual human cells
Working on microscopic pipes only a millionth as wide as a single strand of human hair, Johns Hopkins University researchers have engineered a way to ensure that these tiniest of pipes are safe from the tiniest of leaks.
Leak-free piping, made with nanotubes that self-assemble, self-repair, and can connect themselves to different biostructures, is a significant step toward creating a nanotube network that one day might deliver specialized drugs, proteins, and molecules to targeted cells in the human body. The highly precise measurements are outlined today in Science Advances.
“This study suggests very strongly that it’s feasible to build nanotubes that don’t leak using these easy techniques for self-assembly, where we mix molecules in a solution and just let them form the structure we want,” said Rebecca Schulman, an associate professor of chemical and biomolecular engineering at Johns Hopkins who co-led the research. “In our case, we can also attach these tubes to different endpoints to form something like plumbing.”
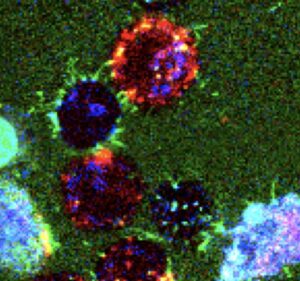
Nanopipes, visible as the thin green lines, attach to cells. Photo credit Schulman Lab/Johns Hopkins University
The team worked with tubes roughly seven nanometers in diameter—about two million times smaller than an ant—and several microns long, or about the length of a dust particle.
The method builds on an established technique that repurposes pieces of DNA as building blocks to grow and repair the tubes while enabling them to seek out and connect to specific structures.
Previous studies have designed similar structures called nanopores. Those designs focus on the capability of DNA nanopores to control the transport of molecules across lab-grown lipid membranes that mimic a cell’s membrane.
But if nanotubes are like pipes, nanopores are like short pipe fittings that alone can’t reach other tubes, tanks, or equipment. Schulman’s team specializes in bio-inspired nanotechnology to address these sorts of problems.
“Building a long tube from a pore could allow molecules not only to cross the pore of a membrane that held the molecules inside a chamber or cell, but also to direct where those molecules go after leaving the cell,” Schulman said. “We were able to build tubes extending from pores much longer than those that had been built before that could bring the transport of molecules along nanotube ‘highways’ close to reality.”
The nanotubes form using DNA strands that are woven between different double helices. Their structures have small gaps in the weave. Because of the extremely small dimensions, scientists had not been able to test whether the tubes could transport molecules for longer distances without leaking or whether molecules could slip through their wall gaps.
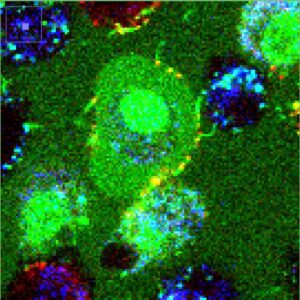
Nanopipes, visible as the thin green lines, attach to cells. Photo credit Schulman Lab/ Johns Hopkins University
Yi Li, a doctoral graduate from Johns Hopkins’ chemical and biomolecular engineering department who co-led the study, performed the nano-equivalent of capping the end of a pipe and turning on a faucet to make sure no water leaks out. Yi capped the ends of the tubes with special DNA “corks,” and ran a solution of fluorescent molecules through them to track leaks and influx rates.
By precisely measuring the shape of the tubes, how their biomolecules connected to specific nanopores, and how fast the fluorescent solution flowed, the team demonstrated how the tubes moved molecules into tiny, lab-grown sacks resembling a cell’s membrane. The glowing molecules slid through like water down a chute.
“Now we can call this more of a plumbing system, because we’re directing the flow of certain materials or molecules across much longer distances using these channels,” Li said. “We are able to control when to stop this flow using another DNA structure that very specifically binds to those channels to stop this transport, working as a valve or a plug.”
DNA nanotubes could help scientists gain a better understanding of how neurons interact with one another. Researchers could also use them to study diseases like cancer, and the functions of the body’s more than 200 types of cells.
Next the team will conduct additional studies with synthetic and real cells, as well as with different types of molecules.
Authors included Johns Hopkins Professor of Physics and Astronomy Brice Ménard, and Himanshu Joshi and Aleksei Aksimentiev from University of Illinois Urbana-Champaign.
The work was supported by: Defense Advanced Research Projects Agency D16AP00147, Department of Energy DE-SC0010426, National Science Foundation DMR-1827346, XSEDE allocation grant MCA05S028, and the Leadership Resource Allocation MCB20012.
Story by Roberto Molar Candanosa and posted on the Hub on September 7, 2022.
Latest Posts
-
Johns Hopkins researchers named to National Academy of Inventors December 12, 2024
-
Breaking Barriers to Better Cellular Therapies December 10, 2024
-
Molecular Morphers: DNA-Powered Gels Shape-Shift on Command November 23, 2024